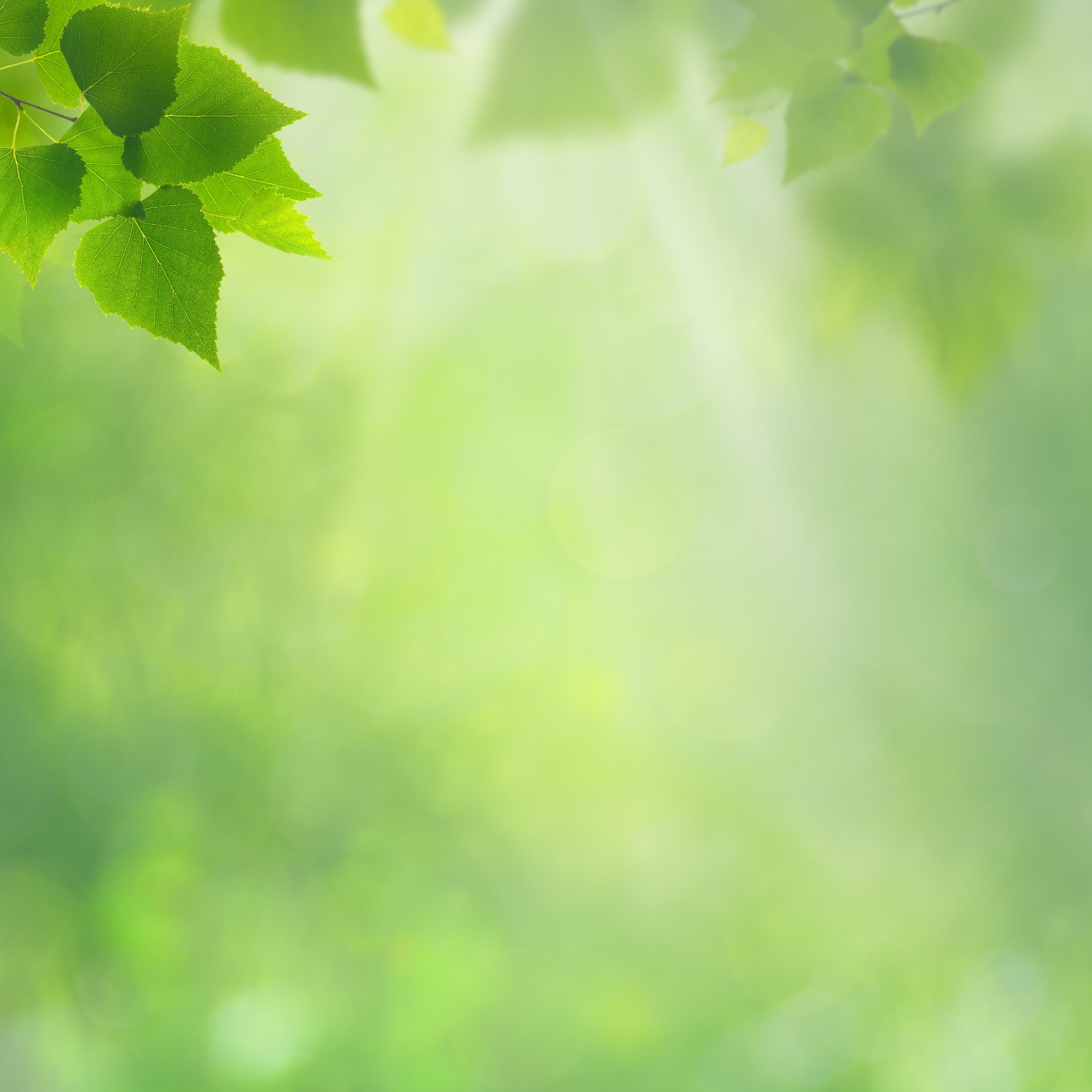
Automatic process is the solution
IAMAS Technologies introducing the novel Photocatalytic Reactor.
This system is not a filter but an advanced water purification system that can eliminate organic pollution, pharmaceutical pollution and heavy metals in the water.
Reclaiming, treating, and reutilizing water is a significant challenge in
many industrial processes, requiring efficient purification technologies
capable of recovering water for productive use.
The novel IAMAS Photocatalytic reactor
treating 2.5 m3/h is developed, combining nanofiltration
synergistically with state-of-the-art photocatalysis, resulting in
applicability extension, operation costs reduction and environmental footprint.
This advanced oxidation technological solution is based on an innovative approach, where active layers and reactor mechanical components are developed using the 3D printing technique.
The optical elements are incorporated into the device with the electronic equipment, the water flow system and the irradiation sources, following a versatile design that permits low-cost manufacture with parallel operational optimization and scale-up ability.
Several operation scenarios and process options were examined concerning feedwater quality, essential material properties and parameters (system productivity, liquid velocity, water permeability, solute rejection efficiency, irradiation intensity) to minimize capital cost and energy demand.
Software development was also implemented, with servicing control of the reactor, data handling and storage linked to validation and demonstration activities.
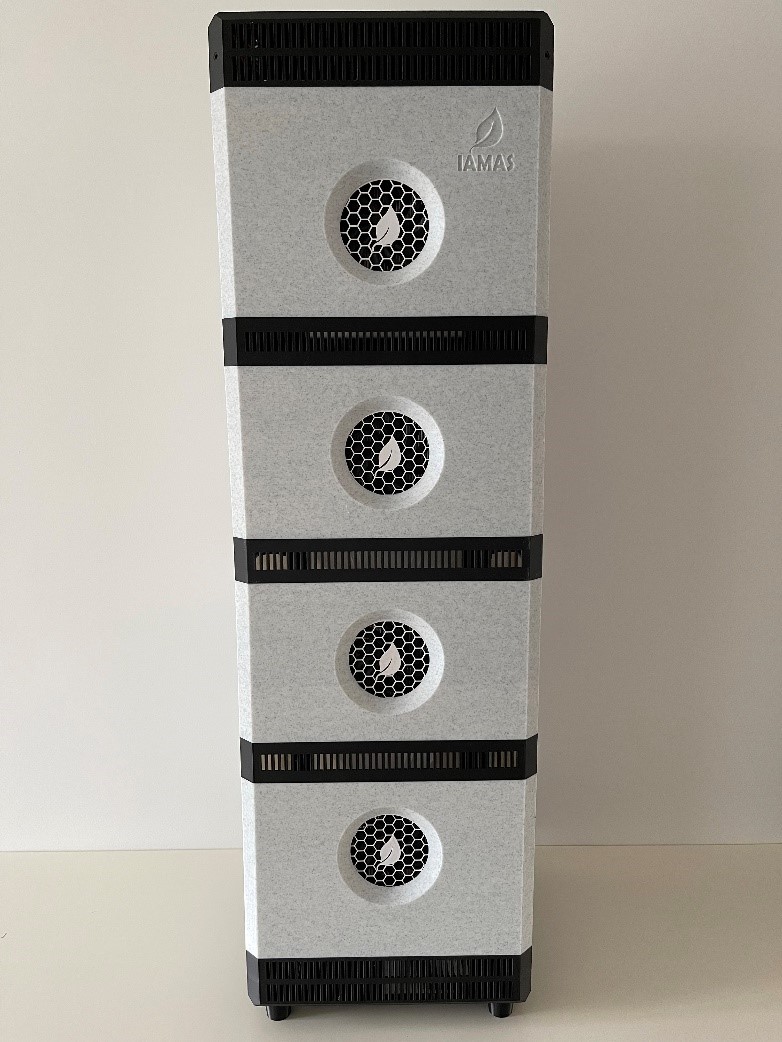
To develop the system, we chose not to use traditional design methods as we did not use conventional manufacturing methods to produce the system parts. We use alternative design and manufacturing ways because conventional manufacturing methods can not make crucial parts of the reactor (lattice and microchannel frames).
The system is designed especially for 3D printing technology manufacturing. This way, we reduce manufacturing costs and save energy and raw materials. Totaly, 100% of the system parts are manufactured and assembled in-house in the United Kingdom at the IAMAS facilities using 3D printing equipment, techniques and materials.
An essential
innovation point is the system's operation through the user-friendly web
interface platform.
We choose to go further to implement IoT (Internet of Things) in the system. We
decentralise the control system by using cloud services and web platforms to operate
and monitor the water purification system.
System Configuration
The system has two
units: the Control and the Photocatalytic Reactor units.
The Control unit is responsible for distributing the water between the
Photocatalytic units, managing the water purification process and measuring the
water quality simultaneously. Also, the control unit contains the communication
modules to synchronise the photocatalytic process between the Photocatalytic
units.
The system can handle multiple photocatalytic units using only a single control
unit, and we can scale the method according to the customer's needs.
The photocatalytic units
can operate in series or parallel according to the water flow demand and the
water pollution.
As an option, we can automatically program the system to arrange the
photocatalytic modules from series to parallel connections.
The photocatalytic unit can operate semiautomatic in stand-alone mode for small
installations without implementing the water quality control modules.
System Specifications
Device dimensions
Length: 280mm - Width: 280mm
- Height: 920mm
Weight: approx 12Kg (Dry)
Power
Power input: 110/220/380VAC 50/60Hz single or three phases adjustable according
to the region and the setup size.
Power consumption: Continuous 280W, Maximum peak of 320W per reactor unit.
LED drivers: DC to DC high power LED efficiency 96%
Connectivity and operation
The system connects to the Internet.
The communication with the system can be through wifi, using the Internet of
things web browser platform.
Other
Water inlet-outlet made through a fast connect plug system.
Water flow range, min 0,25lit/min, nominal 1,4 lit/min, maximum 1,65 l/min.
Water pressure operation from 0.3 to 0.9 bar.
System operating temperatures are 4C to 40C - Input wastewater operating
temperatures range are 4C to 35C.
Active photocatalytic surface minimum 1,5sqm - maximum 1,68sqm.
Innovations
1) Mask Stereolithography microgeometry 3D printed lattice for increasing the photocatalytic surface aspect ratio.
The Mask Stereolithography microgeometry 3D printed lattice offers a very high aspect ratio substrate for the deposition of any photocatalytic (or other catalytic or adsorbent) material, which leads to significant enhancement of the photocatalytic (or other catalytic or adsorptive) surface, thus concluding to remarkable miniaturisation of the photocatalytic reactor (or other catalytic reactor or an adsorber column).
The lattice with the deposited photocatalyst can be directly exposed to UV radiation or visible light on both sides.
The Mask Stereolithography microgeometry 3D printed lattice replaces ceramic monolith membranes that are expensive and demand significant energy to produce them.
The production method of Mask Stereolithography offers significant advances as a low-weight and durable product, with no scrap material during the production process.
The most considerable success of this design is the total of 240 degrees without a shadow on the six layers offers an ideal substrate for depositing and irradiating thin photocatalytic layers.
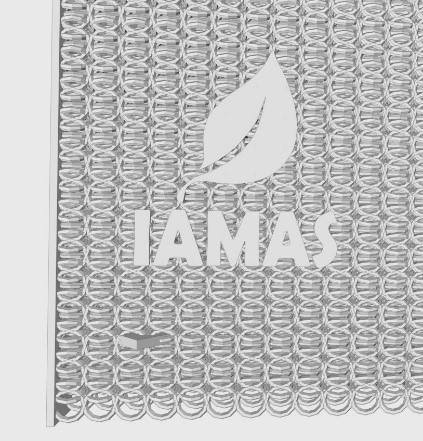
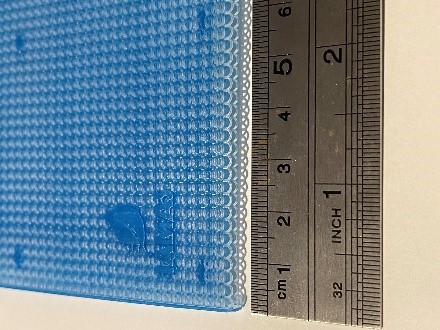
Special Properties
The lattice accepts UV radiation or
visible light on both sides.
This design concentrates
six 4mm thickness multi-angle layers.
Each layer is designed with 0.3mm lines of hexagons and 0.45mm gaps offering
unique geometry to allow the UV radiation to reach three layers of each side.
On the Z-axis, for every step of 32um, we have created horizontal lines of
approximately 8um thickness to increase the coating adhesive and the
photocatalytic surface simultaneously.
We succeeded in this feature by using a unique overexposure MSLA 3D printing technique concerning microstructure patterns on the printing object; we also had the opportunity to discover and improve our skills using this technique during the experimental microstructure prints on this project.
Today the microstructure lattice is a considerable product for commercial and research proposes.
2) 3D-printed micro-fluid channel frames for accurate fluid control.
The intelligent design of a 3D printed micro-fluid channel frame allows unimpeded fluid flow, limiting the pressure drop even at high flow rates and allowing for more efficient contact at the fluid/solid interface.
The Mask Stereolithography 3D printed production method can apply unique multi-channel fluid micro pipes so we can convey the water to the exact point, increasing the system efficiency instantaneously.
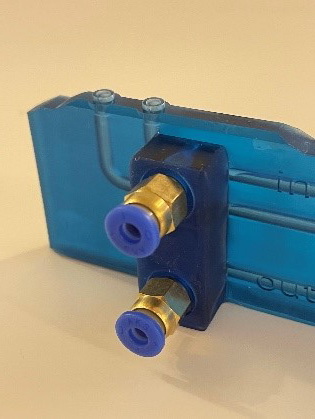
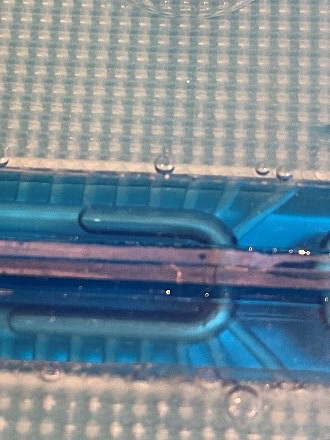
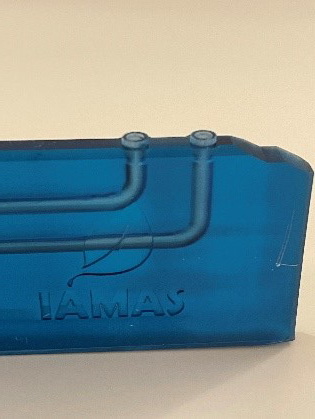
By applying this innovative technology, we can ensure that the water flow has the exact characteristics of flow and pressure inside the irradiated area of the reactor photocatalytic node.
3) A unique 3D printed direct irradiation system equally spreads the UV of visible light to
the photocatalytic surface.
The 3D printed direct radiation system enhances the utilisation of the produced photons (Quantum effect) and reduces the amount of wasted energy from UV sources to the minimum.
The specific geometry of the radiation system creates an illuminable room between the source and the illuminable surface. The direct irradiation system reflects and re-reflects the UV or visible light equally to the photocatalytic surface.
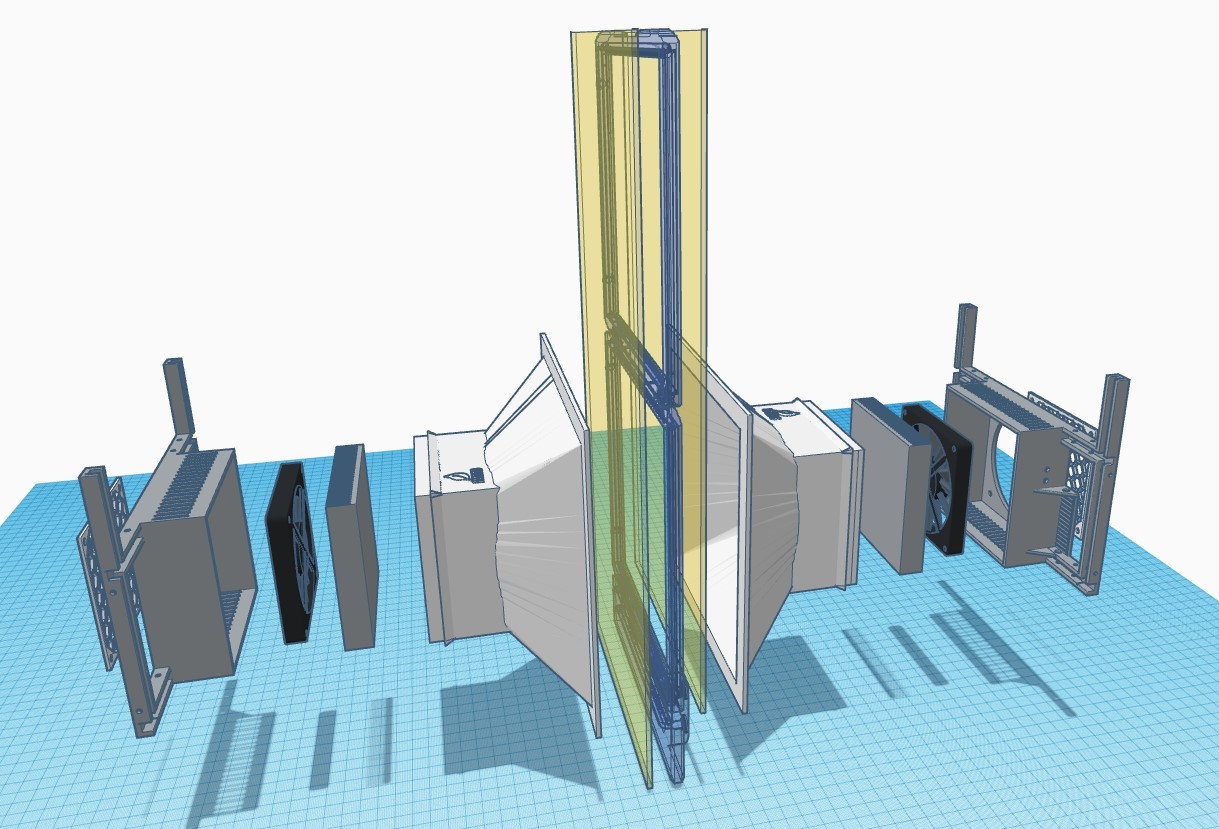
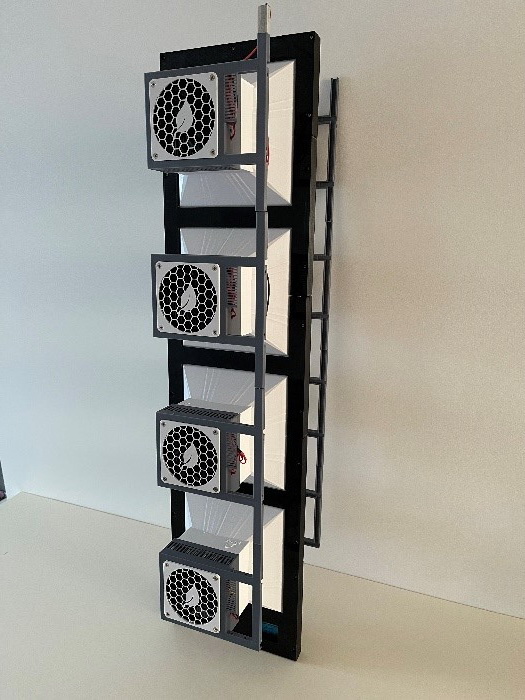
By creating and applying this technology to the Photocatalytic Reactor, we succeed in not over-irradiating areas on the photocatalytic surface, saving energy simultaneously.
4) CO2
sensor
for detecting the CO2
levels in the water.
The CO2 sensor detects the levels of the CO2 gas that dilute to the water during the complete or partial photocatalytic oxidation of organic pollutants.
A significant sensor detects and
monitors the mineralisation efficiency of the photocatalytic process.
The sensor system uses high frequencies to evaporate the water samples and release trapped CO2.
During
the process, the evaporated water (mist) is diverted to the cooling tower,
forcing the gases into the CO2 sensor chamber.
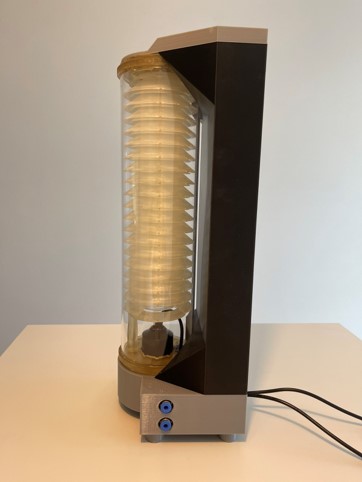
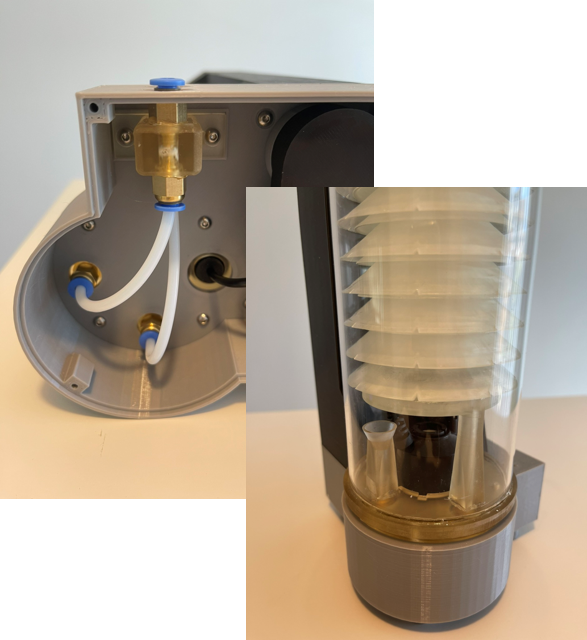
The sensor simplifies the procedure of evaluating the mineralisation efficiency of photocatalytic processes, which is implemented by Total Organic Carbon analysis. This method usually requires sampling in the lab, whereas online TOC equipment is costly.
Regarding the waste management solutions, we have focused on the following innovative technologies:
The philosophy of our proposals is based on new innovative technologies with the aim of creating more attractive and efficient investments in this fields.
IAMAS is a trading name of IAMAS Technologies Ltd. The company IAMAS Technologies Ltd is registered in England and Wales with company number 11842459, the Registered office address is on 269 Farnborough Road Farnborough, London. Post Code: GU14 7LY.